The study of RNAs that do not produce proteins, so-called noncoding RNAs, has been an active area of research for many years. Recently, new kinds of non-coding RNAs have been described that have poorly defined activities. Circular RNA (circRNAs) are one of these more enigmatic biomolecules. They are formed when the 5′ head and 3′ tail of a messenger RNA precursor are spliced together. Next-generation sequencing studies have recently shown that circRNAs are abundant and widely expressed in mammals. While other non-coding RNAs have been shown to play critical roles in cancer, the association between circRNAs and cancer is largely unknown. In addition, the degree to which circRNAs are secreted outside the cell has not been well explored.
To study the presence and regulated release of circRNAs during colorectal cancer (CRC) progression, we used three related colon cancer cell lines that differ only in the mutation status of KRAS, an enzyme that acts at the beginning of a wide array of cellular signaling pathways. The parental cell line (DLD-1) contains both wild-type and G13D mutant KRAS alleles, whereas the derivative cell lines contain only a mutant KRAS (DKO-1) or wild-type KRAS (DKs-8) allele (Shirasawa et al. 1993). The G13D mutation locks KRAS into an active state. KRAS mutations occur in approximately 34–45% of CRCs and have been associated with a wide range of tumor-promoting effects (Vogelstein et al. 1988, Wong and Cunningham 2008). We performed deep RNA-Seq analysis of ribosomal RNA-depleted total RNA libraries to characterize circRNA expression in these cell lines and in the exosomes they release. The results from this study were recently published in the journal Scientific Reports (Dou et al. 2016).
Using a unique pipeline developed by our group, we identified hundreds of high-quality candidate circRNAs in each cell line. Remarkably, circRNAs were significantly down-regulated at a global level in the cell lines with mutant KRAS alleles (DLD-1 and DKO-1) compared to wild type (DKs-8), indicating a widespread effect of mutant KRAS on circRNA abundance (see Figure 1). This finding was confirmed in another pair of cell lines. In all of these cell lines, circRNAs were found associated with secreted exosomes, and circRNAs were more abundant there than in cells. Although circRNAs were down-regulated in cell lines with mutant KRAS alleles, it is difficult to conclude that KRAS directly regulates circRNAs. Nevertheless, our analysis did show that down-regulation of circRNAs in KRAS mutant cells was not caused by their increased export to exosomes.
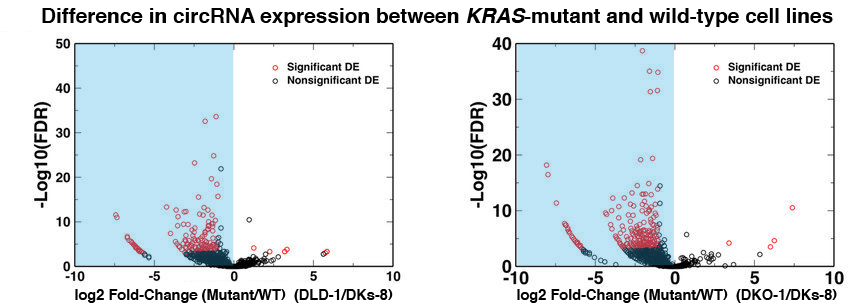
Figure 1. The blue highlight shows that expression of most circRNAs is lower in the KRAS-mutant cell lines than in the KRAS wild-type cell line.
FDR = False Discovery Rate; a higher number indicates a more confident prediction of a difference in expression.
There are complex regulatory mechanisms for expression of both circRNA and the host genes from which they derive. Figure 2 shows that lower expression of circRNA in the mutant KRAS vs. wild-type cell lines was not matched by a similar lower expression of host gene mRNA. We found a similar lack of correlation in circRNA and host gene mRNA expression level in all the exosome populations we studied. These results imply that regulation of circRNAs can occur independent of their host genes, and different regulatory processes might direct secretion of circRNA and host gene mRNA.
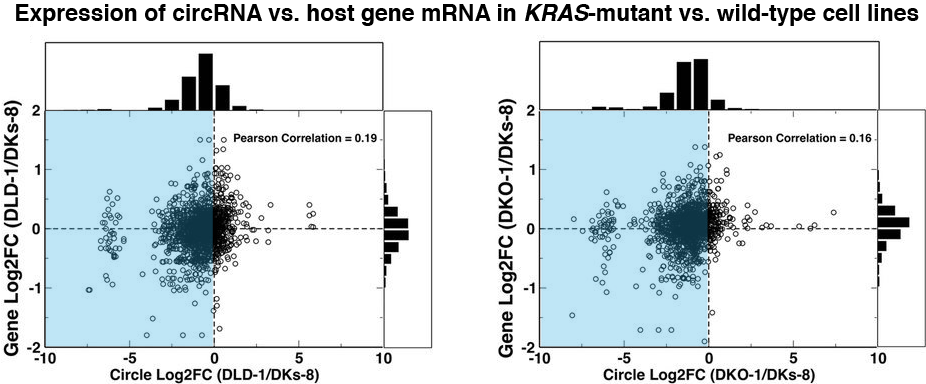
Figure 2. The blue highlight shows that while expression of most circRNAs is lower in the KRAS-mutant than in the wild-type cell line, host gene mRNA expression shows no such pattern.
To further delineate how circRNA biogenesis could be affected by mutant KRAS, we also examined the expression levels of the RNA-editing enzyme ADAR and the RNA-binding protein QKI, which have been reported as circRNA regulators (Ivanov et al. 2015, Conn et al. 2015) (see Figure 3). Here we obtained contradictory results. The level of ADAR was decreased in the KRAS mutant cells; reduced ADAR activity could lead to an increase of circRNAs. QKI was also down-regulated in KRAS mutant cells, which could lead to a decrease of circRNAs.
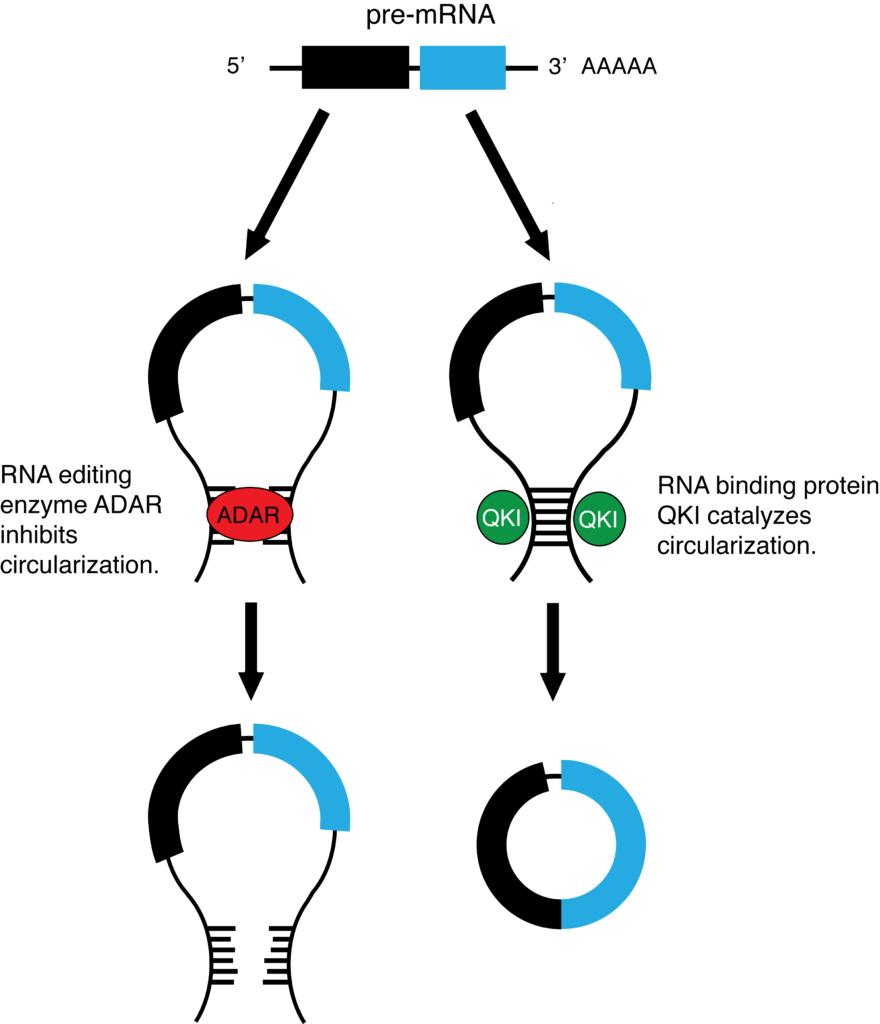
Figure 3. Effect of ADAR and QKI on pre-mRNA circularization
More broadly, we studied the expression levels of all RNA-binding proteins within the RBPDB database (Cook et al. 2011). Six were found to be significantly differentially expressed in KRAS mutant cell lines compared with wild-type KRAS cell lines (ELAVL2, RBMS3, BICC1, MSI1, RBM44, and LARP6). These genes may serve as candidate circRNA regulators. However, our previous work shows that the correlation between mRNA and protein expression level is low for RNA-binding proteins (Zhang et al. 2014), and thus RNA levels for these RNA-binding proteins might not reflect their true protein levels. Further investigation will be needed to precisely define how circRNAs are regulated. Nevertheless, our results show that oncogenic mutations can change circRNA composition in cells and exosomes and suggest that circRNAs may serve as promising cancer biomarkers.
References
Conn, S.J., et al. The RNA binding protein Quaking regulates formation of circRNAs. Cell (2015) 160: 1125-1134. PMID 25768908.
Cook, K.B., et al. RBPDB: a database of RNA-binding specificities. Nucleic Acids Res (2011) 39: D301-D308. PMID 21036867.
Dou, Y., et al. Circular RNAs are down-regulated in KRAS mutant colon cancer cells and can be transferred to exosomes. Sci Rep (2016) 6: 37982. PMID 27892494.
Ivanov, A., et al. Analysis of intron sequences reveals hallmarks of circular RNA biogenesis in animals. Cell Rep (2015) 10:170-177. PMID 25558066.
Shirasawa et al. Altered growth of human colon cancer cell lines disrupted at activated Ki-ras. Science (1993) 260:85-88. PMID 8465203.
Vogelstein, B., et al. Genetic alterations during colorectal-tumor development. N Engl J Med (1988) 319:525-532. PMID 2841597.
Wong, R. and Cunningham, D. Using predictive biomarkers to select patients with advanced colorectal cancer for treatment with Epidermal Growth Factor Receptor antibodies. J Clin Oncol (2008) 26:5668-5670. PMID 19001346.
Zhang, B., et al. Proteogenomic characterization of human colon and rectal cancer. Nature (2014) 513:382-387. PMID 25043054.