The role of extracellular vesicles (EVs) in cancer has recently become a promising area of research. A primary function of EVs is to deliver molecules from a donor cell to regulate cellular processes in a target cell. Little research has investigated what effect departing EVs may have on the donor cell.
In cancer, EVs from tumor cells can deviate from their original purpose. Altering vesicular content could benefit tumors, for example by increasing tumor proliferation or strengthening drug resistance.
Exosomal Packaging
Could donor tumor cells selectively shunt cancer-fighting molecules into secreted vesicles to escape their effects? Teng et al. thought this might be the case. They hypothesized that tumor cells specifically secrete tumor suppressor miRNA, namely miR-193a, into exosomes, a class of EV secreted via the endocytic membrane transport pathway, while oncogenic miRNAs are kept.
To test this theory, they validated miR-193a’s function in a mouse model. Specifically, they found that miR-193a targets Caprin1, a cell-cycle-associated protein, and arrests the cell cycle in phase G1. Thus, secreting miR-193a from the cell in exosomes would restart the cell cycle and enable a tumor cell to proliferate.
After studying miR-193a’s primary function, the authors explored what might facilitate its secretion. They showed that MVP (major vault protein) complexes with miR-193a and that knock-down of MVP leads to higher levels of miR-193a in the cell and less miR-193a in exosomes. They concluded that MVP mediates the sorting of miR-193a into exosomes. They also found that a higher level of MVP in the cell correlates with lower levels of miR-193a and higher levels of Caprin1, indicating that MVP aids in cell proliferation. Lastly, the researchers determined in a mouse model that export of miR-193a by MVP promotes metastasis of colon cancer to the liver.
Figure 1 shows a model of these interactions. In the pre-metastatic cell, miR-193a is freely expressed. In the tumor metastatic cell, MVP has complexed with miR-193a, driving it into exosomes to be secreted.
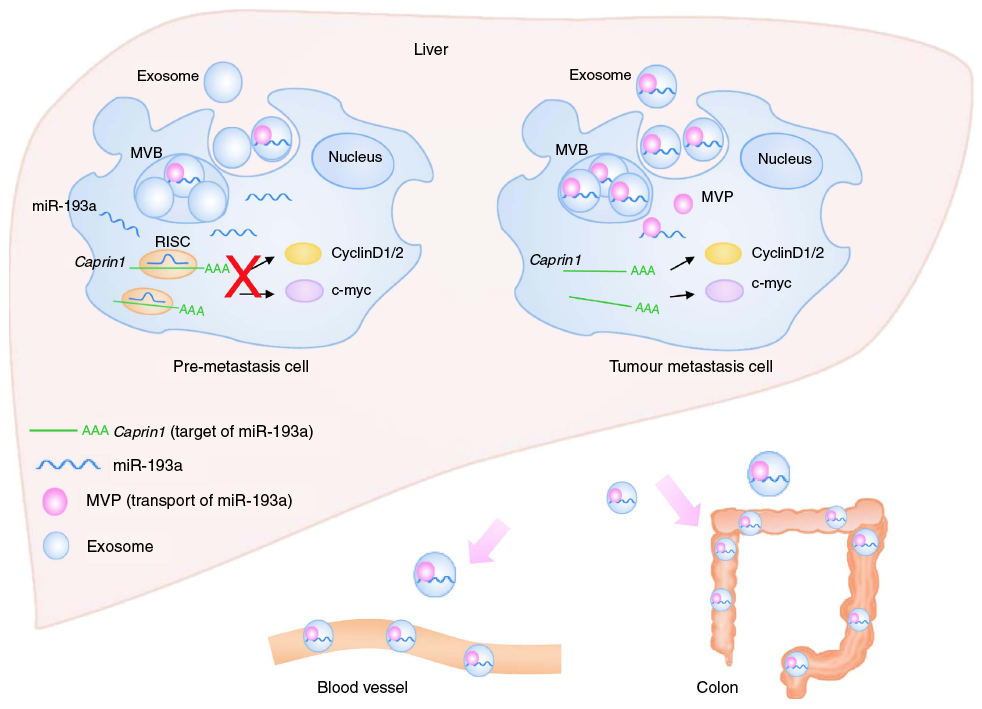
Figure 1: Model for the mechanism of colon cancer metastasis to the liver. Tumor suppressor mir-193a is sorted into exosomes by Major Vault Protein (MVP) and then secreted from the cell.
Cancer Biomarker
This finding implicates exosomal miR-193a as a potential biomarker for colon cancer. Could higher levels of exosomal miR-193a indicate a more aggressive disease? To answer these questions, the authors applied their findings in the clinical setting. Teng et al. examined the livers of mice with metastatic colon cancer, performing an extensive characterization of the levels of tumor suppressive and oncogenic miRNAs in normal and tumor cells and in the exosomes secreted by both. From that extensive analysis, they chose three miRNAs upregulated (miR-193a, miR-126 and miR-148a) and one miRNA downregulated (miR-196b) in exosomes from tumor cells and examined the levels of those miRNAs in exosomes purified from the blood (plasma) of 40 colon cancer patients, 15 with metastasis to the liver and 25 without. They found the same upregulation and downregulation of the 4 miRNAs in the patient population, with higher levels in the population with liver metastasis.
This exciting study identifies potential biomarkers for colon cancer. The two main findings highlight the importance of exosomes in cancer proliferation. First, the authors found that tumor suppressing miRNAs are packed into exosomes, while oncogenic miRNAs remain in the cell. Next, they found that there are higher amounts of tumor suppressing miRNAs in tumor-derived exosomes relative to exosomes from healthy cells. They also found that MVP acts as a mediator of these differences between metastatic and healthy cells. Teng et al. believe that once we develop a dependable method to purify exosomes, scientists may uncover further roles for exosomes in cancer progression.