For researchers who have just begun studying extracellular vesicles (EVs) and their contents, including extracellular RNA, the Extracellular RNA Communication consortium (ERCC) published a protocols decision tree today, designed to help select a set of protocols for isolating EVs and exRNA from several biofluids of interest. Some of the protocols in the decision tree have been developed by ERCC researchers, but many relevant methods were published before the ERCC existed, and the versions on the ExRNA Portal include modifications and comments made by ERCC members in the course of their experiments, and are being periodically updated. In this blog, we introduce the ERCC protocols decision tree and discuss some of the nuanced differences between classes of EV isolation methods, highlighting methods that have existed in the field for some time.
There are several methods and commercial kits available to isolate extracellular vesicles (EVs) and extracellular RNA (exRNA) from human biofluids. Multiple studies have reported on a variety of these methods, but to date there is not one method or kit that suits all studies. The type of biofluid, the sample volume, and the fraction of exRNAs of interest are some of the criteria used to determine what method should be used for a particular study. Here, we have compiled a list of methods and kits most widely reported in the literature for isolation of EVs, exRNA or other components of biofluids. Broadly speaking, all methods can be classified into five categories (see Table), the most widely used being ultracentrifugation.
Drawbacks of ultracentrifugation include the need for expensive instrumentation (ultracentrifuges and rotors) and the belief that the EV population after ultracentrifugation will be contaminated with cell-free DNA and proteins. Depending on the speed and time of ultracentrifugation, some ribonucleoprotein (RNP) and lipoprotein (LPP) complexes may also sediment. After ultracentrifugation, some researchers further purify the EV population using density gradients or size exclusion chromatography. Sucrose gradients have been used widely for several years but are being replaced more recently by iodixanol (OptiPrep) gradients because some groups have reported that sucrose may inhibit the biological effects of EVs, while EVs prepared with OptiPrep better retain their biological activity.
Size exclusion chromatography is also widely used and is suitable for fractionation of sedimented EVs, as well as unprocessed biofluids. Recently Izon introduced a commercial kit to speed up this method, with relatively good results. Size exclusion chromatography yields a very clean population of EVs with the drawback being loss of EVs during the multi-step purification process.
The first commercial EV isolation kit (ExoquickTM) was launched on the market about six years ago, and is based on the principle of polyethylene glycol/sodium chloride precipitation, which has long been used for concentration of viruses. Since that time, several other kits using a precipitation strategy were launched by other manufacturers. Each of these kits (SBI, Life Technologies, Norgen Biotek, and Exiqon) have slightly different proprietary approaches to EV precipitation. Drawbacks to EV precipitation kits include co-precipitation of other unwanted molecules found in the biofluids and the difficulty of isolating EVs from large volumes of starting material. Ultrafiltration (e.g. using Millipore Amicon filters) is often used by researchers to concentrate large volumes, either before or after EV purification.
Filtration based methods which isolate specific size ranges of EVs can also be performed using commercially available devices. In addition, several kits and protocols for affinity purification have been developed by biotechnology companies and academic research laboratories. Antibody-based affinity methods (ExoCap, Microfluids, µNMR), and heparin-coated agarose or magnetic beads have been shown to bind subpopulations of EVs from cell culture media, plasma and serum efficiently. Another affinity kit, the METE kit, includes a proprietary peptide that, according to the manufacturer, binds to heat shock proteins found on the surface of the plasma membrane, suggesting a possible method to enrich for EVs with high levels of heat shock proteins. All of these methods yield a pure sample of EVs and can be scaled up, although scale-up costs can be significant, particularly in the case of antibody-based methods. These methods are limited to isolating a subset of EVs that express a specific antigen. For researchers who are interested in targeting a specific population EVs that displays one of these antigens, this may be a good option. However, at this point, for most cases, the biology is unclear on the diversity of EVs released by cells. Therefore, one antibody, or a pool of three to four antibodies, may not isolate all relevant EVs present in the sample.
ExoRNeasy isolates EVs based on their affinity to a proprietary membrane. ERCC members using this kit have reported that it can efficiently separate EVs (they bind to the membrane with high affinity) from other exRNA-containing particles, such as RNP complexes, which can be collected in the flow through, so this kit offers the extra advantage of efficiently separating EVs from RNP complexes. The ExoRNeasy/exoEasy kit yields a pure EV population and can isolate EVs from a volume as low as 200 μL or as high as 4 mL of plasma or serum with one loading per column, and up to 100 mL of cultured media per column by loading the column 3-4 times. Larger volumes require loading multiple filters.
Two kits available on the market are based on a two-step procedure where the sample is first either filtered (e.g. PureExo) or concentrated (e.g. Exo-Spin) and then resuspended and allowed to bind to proprietary beads. Intact EVs are then eluted in PBS and can be used for a variety of downstream assays. These kits do not offer feasible approaches to scale up to larger volumes.
Many other combinations and variations on these methods have also been reported in the literature, and this list is not meant to comprehensively encompass all reports in the field. It is a simple overview of the major classes of EV and RNA isolation methods present in the EV isolation field.
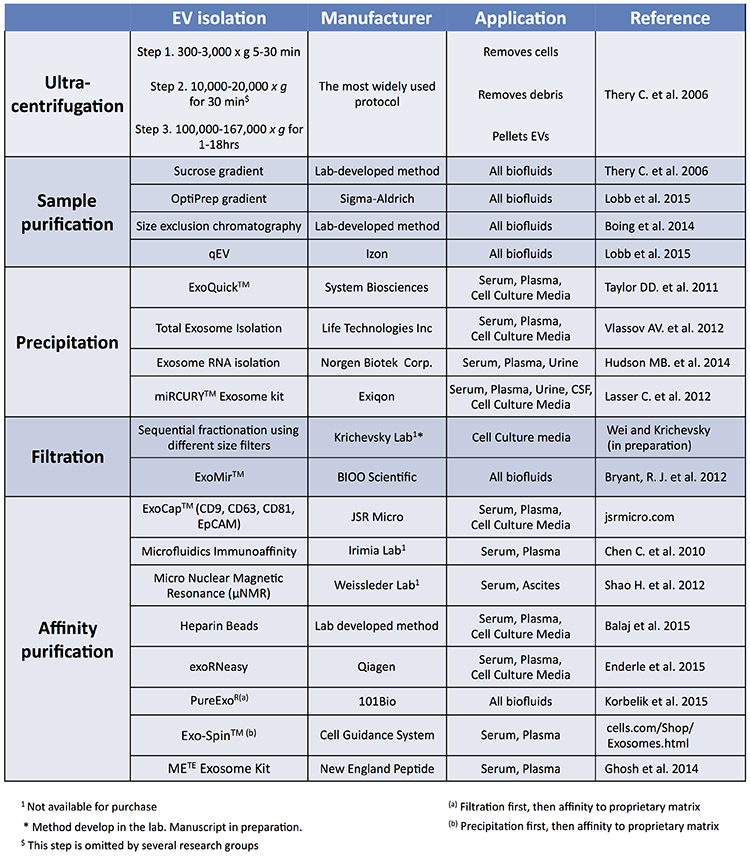