(This blog first appeared as a press release from Ohio State University.)
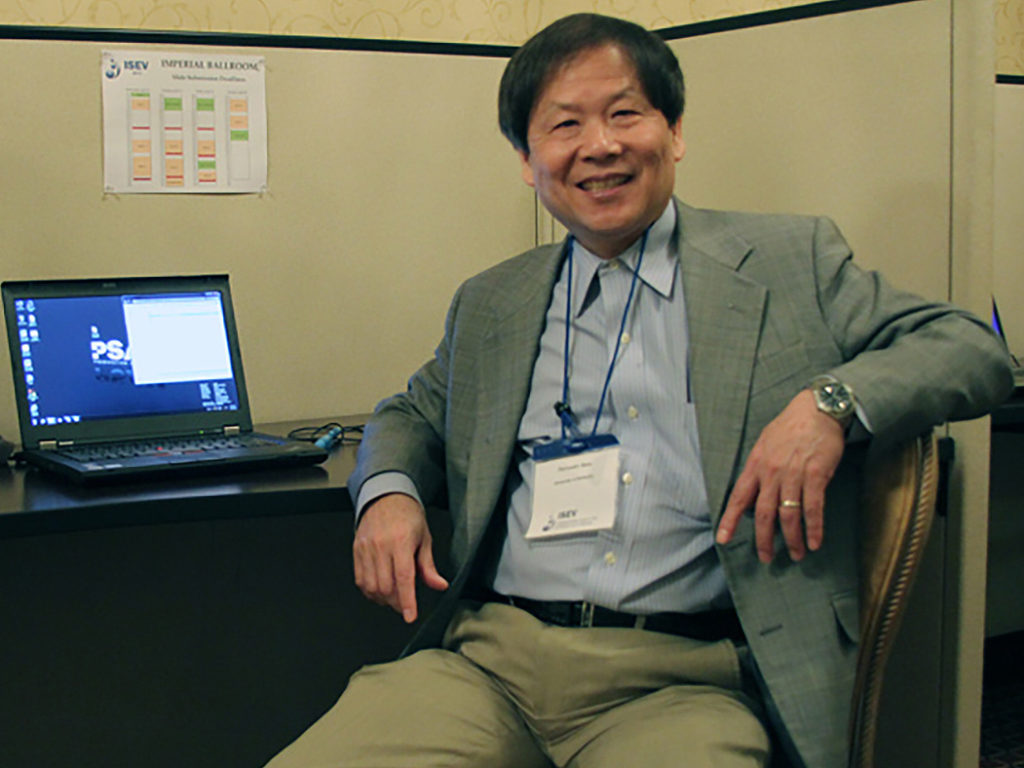
Principal investigator Peixuan Guo, PhD, Sylvan G. Frank Endowed Chair professor of the OSU College of Pharmacy and a member of the OSUCCC – James Translational Therapeutics Program.
- Therapies based on RNA, such as small interfering RNA, hold great promise for cancer treatment but delivering these agents to their targets in cancer cells has been a problem.
- A new study shows that attaching antibody-like RNA nanoparticles to microvesicles can deliver effective RNA therapeutics specifically to cancer cells.
- The researchers are now working to adapt the technology for use in the clinic.
Columbus, Ohio – A new study shows that attaching antibody-like RNA nanoparticles to microvesicles can deliver effective RNA therapeutics such as small interfering RNA (siRNA) specifically to cancer cells. Researchers used RNA nanotechnology to apply the RNA nanoparticles and control their orientation to produce microscopic, therapy-loaded extracellular vesicles that successfully targeted three types of cancer in animal models.
The findings, reported in the journal Nature Nanotechnology, could lead to a new generation of anticancer drugs that use siRNA, microRNA and other RNA-interference technologies.
The study was led by researchers at Ohio State’s College of Pharmacy; the Ohio State University Comprehensive Cancer Center – James Cancer Hospital and Solove Research Institute (OSUCCC – James).
“Therapies that use siRNA and RNA interference technologies are poised to transform cancer therapy,” says the principal investigator Peixuan Guo, PhD, Sylvan G. Frank Endowed Chair professor of the College of Pharmacy and a member of the OSUCCC – James Translational Therapeutics Program. “But clinical trials evaluating these agents have failed one after another due to the inability to deliver the agents directly to cancer cells in the human body.”
Guo noted that even when agents did reach and enter cancer cells, they were trapped in internal vesicles called endosomes and rendered ineffective.
“Our findings solve two major problems that impede these promising anticancer treatments: targeted delivery of the vesicles to tumor cells and freeing the therapeutic from the endosome traps after it is taken up by cancer cells. In this study, cancers stopped growing after systemic injection of these particles into animal models with tumors derived from human patients.” Guo says. “We’re working now to translate this technology into clinical applications.”
Guo and his colleagues produced extracellular microvesicles (exosomes) that display antibody-like RNA molecules called aptamers that bind with a surface marker that is overexpressed by each of three tumor types:
- To inhibit prostate cancer, vesicles were designed to bind to prostate-specific membrane antigen (PSMA);
- To inhibit breast cancer, vesicles were designed to bind to epidermal growth factor receptor (EGFR);
- To inhibit a colorectal cancer graft of human origin, vesicles were designed to bind to folate receptors.
All vesicles were loaded with a small interfering RNA for down-regulating the survivin gene as a test therapy. The survivin gene inhibits apoptosis and is overexpressed in many cancer types.
Key findings include:
- Vesicles targeting the prostate-specific membrane antigen completely inhibited prostate-cancer growth in an animal model with no observed toxicity.
- Vesicles targeting EGFR inhibited breast cancer growth in an animal model.
- Vesicles targeting folate receptors significantly suppressed tumor growth of human patient-derived colorectal cancer in an animal model.
“Overall, our study suggests that RNA nanotechnology can be used to program natural extracellular vesicles for delivery of interfering RNAs specifically to cancer cells,” Guo says.
Funding from the National Institutes of Health/National Cancer Institute (grants TR000875 and CA207946, CA186100, CA197706, CA177558 and CA195573) supported this research.
Other researchers involved in this study were Fengmei Pi, Daniel W. Binzel, Zhefeng Li, Hui Li, Farzin Haque, Shaoying Wang and Carlo M. Croce, The Ohio State University Wexner Medical Center; Meiyan Sun and Bin Guo, University of Houston; Piotr Rychahou and B. Mark Evers, University of Kentucky; and Tae Jin Lee, now at University of Texas.
About the OSUCCC – James
The Ohio State University Comprehensive Cancer Center – Arthur G. James Cancer Hospital and Richard J. Solove Research Institute strives to create a cancer-free world by integrating scientific research with excellence in education and patient-centered care, a strategy that leads to better methods of prevention, detection and treatment. Ohio State is one of only 49 National Cancer Institute (NCI)-designated Comprehensive Cancer Centers and one of only a few centers funded by the NCI to conduct both phase I and phase II clinical trials on novel anticancer drugs sponsored by the NCI. As the cancer program’s 308-bed adult patient-care component, The James is one of the top cancer hospitals in the nation as ranked by U.S. News & World Report and has achieved Magnet designation, the highest honor an organization can receive for quality patient care and professional nursing practice. At 21 floors with more than 1.1 million square feet, The James is a transformational facility that fosters collaboration and integration of cancer research and clinical cancer care.